It is a division of analytical chemistry that deals with determining the structure of the analysed chemical compound, including the atoms it contains and the way they are interlinked. It covers a whole set of techniques that enable the acquisition of such information. We often carry out a full range of structural analyses if we synthesise a new compound, in order to confirm the possible structure, but also to confirm the products assumed during the chemical reaction. The most reliable techniques are spectral analyses, which are such analyses that give an outcome in the form of the spectra of particular relationships. This kind of graphic representations makes it possible to read out the types of elements existing in the sample, the link energies, and the system of molecules and their atoms. A great advantage of spectral analyses is the fact that even a trace amount of the substance is enough to conduct an experiment. The most precise techniques used in structural analysis are the following: nuclear magnetic resonance spectroscopy, infrared spectroscopy, UV-Vis spectroscopy, X-ray crystallography, mass spectrometry, Raman spectroscopy, and microanalysis.
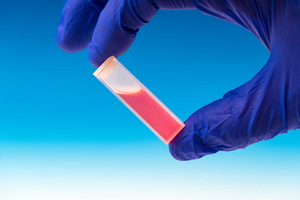
Nuclear magnetic resonance (NMR) spectroscopy
During the measurement, we use electromagnetic radiation in the range of 60 to 900 MHz, which carries a small portion of energy. This enables the excitation of transitions between different levels which have a low energy barrier. This is characteristic of quantum states, which are closely linked to magnetic properties of the nuclei of the atoms that build chemical molecules. An important aspect of those properties is the spin, which is macroscopically reflected in physics in the form of angular momentum. If we take, for example, a proton with a positive charge, which also has a spin, the motion of the charge is totally ordered, which in turn reflects a macroscopic flow of current. This is always accompanied by the formation of a magnetic field, and the proton itself becomes a sort of a magnet. Where there is no external magnetic field, the spatial orientation of such a dipole has no interrelations; it is free. However, if we apply an external magnetic field, the dipoles will position themselves along the field to form an ordered system. By supplying an appropriate amount of energy, it is possible to turn the spin to specified positions relative to the lines of the external magnetic field. This is only possible if the magnetic quantum number of that molecule equals -½ or ½ . For such quantum states, it is possible to excite energy transitions with the use of electromagnetic radiation with the appropriate energy. For nuclei that have more nucleons, we can also observe relationships between the structure of an atomic nucleus and its quantum number.
- The atomic nuclei that contain an even number of protons and neutrons are characterised by the quantum spin number (I) equal to 0, so also the spin value can only equal 0. In consequence, it is impossible to excite energy transitions. Such a situation exists in several isotopes being important to the analyses of organic chemistry: 12C and 16 We cannot obtain NMR spectra for such isotopes.
- The nuclei built of an even number of nucleons of one type and an odd number of nucleons of another type have a quantum spin number of ½ or its multiple. Such nuclei include 13C, 15N, 19F and 31P, and they behave similarly to the protons. These isotopes, for which we can obtain the NMR spectrum, are extremely valuable to analytical chemistry.
- If a nucleus contains an odd number of protons and neutrons, the quantum spin number is equal to the total quantity. The most important example is the nucleus of deuterium 2 This makes it possible to use it in solvents, and consequently its resonance signal is a signal of field stabilisation and scale calibration for NMR spectrometers.
Spectral interpretation allows us, depending on the type of NMR, to determine valuable information, for example the 1H NMR shows the quantity and type of the present groups of protons and suggests the structural fragments. The spectrum of 13C NMR will present signals corresponding to carbon atoms in points characteristic of different groups.
Infrared (IR) spectroscopy
This technique makes it possible to observe the oscillation spectra of molecules in the range from 4000 to 400 cm-1. An excitation that allows for forming a spectrum is related to the vibration of bonds and the change of angles between bonds, both within and outside the plane. The spectrum shows the dependence of transmittance on the wave number, and the lower the transmittance, the more intensive the absorption. The absorption bands visible in the spectrum are specific to the vibration of bonds, depending on the spectral range:
- an area below 1500 cm-1 may include extending vibrations of C-O, C-N and C-C as well as deforming vibrations,
- the range of 2000–1500 cm-1 includes extending vibrations of C=O, C=N, C=C double bonds,
- extending vibrations of triple bonds are visible within the range of 2500–2000 cm-1,
- the range of 4000–2500cm-1 shows extending vibrations of O-H, N-H and C-H bonds.
Spectral interpretation makes it possible to identify the functional groups present in the structure and to determine the general structure of the compound, including its aromaticity and possible saturation.
UV-Vis spectroscopy
This method uses an electromagnetic irradiation spectrum in the range of 200 to 780 nm. Its theoretical basis is the energy absorption in the ultraviolet area, which is equivalent to electron transitions from the basic to the excited state. It is quantized, which means it exactly corresponds to the difference between energy levels. The smaller the difference, the longer the wavelength of the absorbed radiation. The spectrum is composed of absorption bands and presented as the relationship of absorbance (A) to wavelength (λ). The UV-Vis spectroscopy is usually used for confirming or excluding the presence of chromophores, i.e. groups of atoms capable of absorbing electrons. Sometimes it also helps to determine the relative position of those groups.
Mass spectrometry (MS)
This method enables us to examine substances with the use of the spectrum of the masses of atoms and molecules contained in the substance. They undergo ionisation in the gaseous phase and then are separated based on the mass-to-ion charge ratio. Having the spectrum derived in MS analysis, we can determine the value of masses and the relative content of ingredients of the tested substance. The method also makes it possible to identify various fragments of the structure. Molecule fragmentation is performed by consecutive decompositions of bonds with the lowest energy. The intensity of the resulting ion beams depends directly on the durability of the cations being formed and on the speed of the subsequent fragmentation stages. The use of that technique is aimed at determining the molecular mass, chemical composition and structural design of particles, substance purity, and contaminant identification. Its advantages include accuracy, a wide range of applications, and a resolution at the level of several atomic mass units.