Dissociation, in chemical context, means the decomposition of a substance into other molecules. The word comes from the Latin term “dissociatio”, meaning “separation”. In scientific nomenclature, dissociation has various definitions, as it also has its specific meaning in psychology. But let us focus on what dissociation means in chemistry. Depending on the course of the process and the substance used, we can distinguish four different types of dissociation. The most popular one is electrolytic dissociation, although thermal dissociation is equally important.
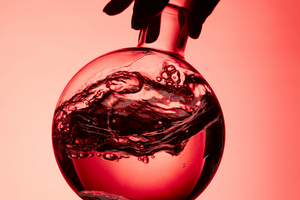
Thermal dissociation
The process consists in the decomposition of molecules into smaller molecules or into atoms, under the influence of temperature. The most important relationship is the fact that the temperature used for dissociation may be lower if the compound includes weaker chemical bonds. The ones with a higher energy necessary for decomposition require a higher temperature. A common example of thermolysis (thermal dissociation) is the caramelization of sucrose, which occurs at 160oC.
Electrolytic dissociation
Discovered in 1887 by the Swedish chemist Svante Arrhenius, it is somewhat more complex compared to thermal dissociation. Electrolytic dissociation consists in the decomposition of a substance, which occurs when a solvent (usually water) affects freely moving ions. Arrhenius has proved in his research that the ions produced by such dissociation cause the solution to conduct electricity. This was the foundation to develop the classification of chemical substances into electrolytes (solutions capable of conducting electric current) and non-electrolytes (solutions not capable of conducting electric current).
Electrolytes
The term “electrolytes” has two meanings –from the technical point of view, it is any substance that can ionically transfer a charge between electrodes. As a result, it may ionically conduct electric current. The other, more chemical meaning of that term refers to substances which undergo electrolytic dissociation as a consequence of melting or dissolution. Such solutions (most often aqueous) also conduct electricity. Examples of such electrolytes include ionic compounds, such as sodium chloride (table salt), but also non-ionic compounds, for instance hydrochloric acid or potassium hydroxide. Given their specific properties, electrolytes can be called electrical conductors. These may be divided into the class 1 conductors (metals, their alloys, and carbon) and class 2 conductors (solutions of bases, acids and salts).
The mechanism of electrolytic dissociation
In a solvent whose dielectric constant is high (e.g., 81 for water), the forces of mutual attraction between ions in a crystalline network are considerably reduced. This is when the ions break off, which causes the substance to dissolve and secrete freely moving ions into the solution. Such processes also occur due to the interaction between ions and water molecules, which is a consequence of a polar structure of H2O. According to Nekrasov, the mechanism of action of water during the decomposition of an electrolyte with an ionic structure is started by the substance’s positive ion, which attracts the opposite pole of the water molecule, at the same time pushing away the positive ion. The opposite pole acts in the same way, and both ions get surrounded by the water molecules they have attracted. Such attraction occurs in both directions between dipolar molecules and ions, which makes the attractive force of ions in the electrolyte much lower. Thus, the mechanism can be divided into four principal stages: the transition of polar into ionic structure, the formation of the ionic structure, and the hydration of ions. The links between the ions and the solvent, produced by dissociation, are called solvates. If water is the solvent, then they are called hydrates.
The hydration shell
It is a shell composed of water molecules existing in the closest proximity of the ion, oriented with their opposite poles. Free, charged poles attract further water layers, until the ion’s attractive force becomes weaker than the molecular motion energy in the solution. The shell facilitates the decomposition of electrolyte molecules into cations and anions, and constitutes a barrier that hinders the re-linking of ions to form molecules.
Dissociation of acids and bases: the law of mass action
Strong acids are the acids which show far-reaching dissociation in an aqueous solution, and weak acids are those which dissociate only to a small extent. If we compare the strength of different acids, we should also take into account a few factors such as the tendency of acid molecules to give away their protons, or the concentration of the substance in question. However, there is a chemical value which does not depend on concentration but excellently characterises individual properties of molecules – the dissociation constant. Deriving the equation for that value should be started by writing the equation for acid dissociation. For an example with a single proton (HX), it will look like this:
If the solution is diluted and the acid is weak, we can apply the law of mass action, which is expressed as follows:
There are two ways to make the presented relationship shorter. The first step is to assume that the water concentration is constant. We can also omit the co symbol, as it expresses a standard concentration equal to 1 mol/dm3. However, it is important to place it in the initial formula and to take it into account when determining the unit. This is because chemical constants are dimensionless values. Meanwhile, all the concentrations used in the formula are expressed in mol/dm3. Then we finally obtain the formula for the dissociation constant:
The relationship expressing the acid dissociation constant (Ka) consists of values determined by experiment, which fall within the range of 10-10 to 108. A popular operation is to use a logarithmic formula, expressed as a negative logarithm of the dissociation constant, designated as pKa:
pKa = -logKa
It is important that while low Ka values represent weak acids, and high values represent strong acids, the pKa changes in the opposite way. Low, usually negative pKa values correspond to strong acids. Positive, high pKa values represent weak acids. When we introduce another concept, the degree of dissociation (α), which is the ratio of the number of moles of the molecules of a given compound, which undergo dissociation (decomposition into ions), to the total number of moles of the molecules of that compound, present in the solution, we can further develop the equation. If we disregard the very low concentration of hydrogen ions formed by water dissociation, and use the equilibrium concentrations of ions and molecules, we may assume that:
Then the dissociation constant can be noted as follows:
In this form, it is the dependency of the degree of dissociation of an electrolyte from its concentration, i.e., Ostwald’s law of dilution. If we analyse very weak electrolytes, the degree of dissociation (α) may be assumed as <<1, and the formula can be simplified to the following:
Acids which contain more than one proton undergo multi-stage dissociation, and we must take all its stages into account. To give an example, for orthophosphoric acid there are three stages:
- H3PO4 + H2O = H2PO4– + H3O+
- H2PO4– + H2O = HPO42- + H3O+
- HPO42- + H2O = PO43- + H3O+
Each stage has its own equilibrium constant, which can be noted analogously to the first one:
Dissociation constants are tabulated, both for hydracids and for oxyacids.
Acid | Ka | pKa |
HF | 6,3·10-4 | 3.2 |
HCl | 1·107 | -7 |
HNO3 | 27.54 | -1.44 |
H3PO4 | 6.9·10-3 | 2.16 |
Table 1. Example values of dissociation constants for acids.
It is similar for the stabilisation of the equilibrium of bases during dissociation. Considering the example rule B, the equation can be noted as follows:
Then, if we wish to derive a dissociation constant for a base, using the law of mass action and assuming that the water concentration in the diluted solution is virtually constant, we obtain the following formula:
The stronger the base, the higher the dissociation constant and the lower the pKb value, whereas the lower the dissociation constant and the higher the pKb value, the weaker the base.
Base | Kb | pKb |
Hydrazine | 3·10-6 | 5.5 |
Ammonia | 1.78·10-5 | 4.75 |
Methylamine | 5.0·10-4 | 3.3 |
Table 2. Example values of dissociation constants for bases.
What determines the strength of acids
If we know the expressions of dissociation constants for particular acids, we can make a few true assumptions:
- The strength of a hydracid increases along with the increasing atomic number of the element linked with the hydrogen atom.
- The strength of a hydracid declines along with the increasing electronegativity of the element attached to the hydrogen atom. This may be misleading, as in theory the increasing electronegativity should favour the abstraction of protons.
- The size of the anion formed during dissociation affects the dissociation constant to a greater extent than electronegativity.
Self-dissociation of water
An interesting example of dissociation is the decomposition of water molecules. Since they are capable both to draw protons and to give them away, pure water undergoes self-dissociation to a small extent, as per the following equation:
H2O + H2O ↔ H3O+ + OH–
Like for acids and bases, it is also possible to apply the law of mass action, which includes equilibrium concentrations of non-dissociated water as well as its anions and cations:
It turns out that the concentrations of H3O+ cations and OH– anions are so small compared to non-dissociated water that even their alteration practically does not change the concentration of non-dissociated water. This makes it possible to assume that value to be constant and to convert the equation to the following form:
Kw = cOH– · cH3O+
It is a particularly important aspect in the context of chemical computations, and such a constant is referred to as the ionic product of water. It is also often used as a negative logarithm, designated as pKw. This is equal to –logKw. Based on the equation, if the ionic equilibrium of water is disturbed as a result of supplying H3O+ ions, then in order to maintain constancy of the ionic product we must reduce the concentration of OH– ions. Similarly, an excess of OH– ions reduces the concentration of cations. The product of these concentrations must be constant. Experiments have shown that Kw at a temperature of 298K is 10-14. Therefore, we can also note the equation valid for pure water as follows:
Dissociation is also related to the solution’s pH value. Solutions with equal concentrations of H3O+ cations and OH– anions show a neutral pH. In acidic solutions the concentration of cations is higher than 10-7 mol/l, while in alkaline solutions it is lower than 10-7 mol/l.